Brain sample preparation
The brain samples used in this study were donated by the Department of Anatomy, Fukushima Medical University. Sixty cerebral hemispheres from anonymous donors were used. Formalin (37% formaldehyde aqueous solution;Koei Chemical Co., Ltd., Tokyo, Japan) was used for brain fixation. After death was confirmed, diluted 4% formalin was flushed through the donors’ femoral arteries. Within 48 h after death, brain samples were harvested and submerged in 10% formalin for 3 days and then in diluted 4% formalin for one to 3 months. These formalin-fixed samples were then allocated to one of ten freezing protocol groups:formalin-fixed (P1), 1-week continuous freeze (P2), 2-week continuous freeze (P3), 3-week continuous freeze (P4), 4-week continuous freeze (P5), 5-week continuous freeze (P6), two cycles of 1-week continuous freeze separated by a 6-hour thaw period (P7), three cycles of 1-week continuous freeze separated by 6-hour thaw periods (P8), four cycles of 1-week continuous freeze separated by a 6-hour thaw periods (P9), and five cycles of 1-week continuous freeze separated by 6-hour thaw periods (P10). Six hemispheres were allocated to each protocol. For freezing, each hemisphere was wrapped in gauze and cellophane film and stored at −5°C. For thawing, the hemispheres were transferred into a bucket and placed under running tap water for 6 hours.
Following thawing, each hemisphere underwent slicing into six 5-mm sections, with three positioned anteriorly and three posteriorly to the central sulcus (Fig.1A). Subsequently, within each of these six slices, three distinct regions were delineated for further preparation and analysis, predominantly encompassing U-fibers and long fibers located at the (1) ventral operculum, (2) temporal lobe, and (3) dorsal operculum (Fig.1B). These three designated regions were then embedded in paraffin, with each subsequently sliced into 6-μm sections and subjected to hematoxylin-eosin (H&E) staining, as illustrated in Fig.2. The resultant images were digitally scanned using the Keyence BZ-X700 digital microscope (Keyence Corporation, Osaka, Japan) and transferred to image processing software (Fig.3A). These digitized images served as the basis for subsequent analyses, including the assessment of gap ratio and eccentricity (further described below).
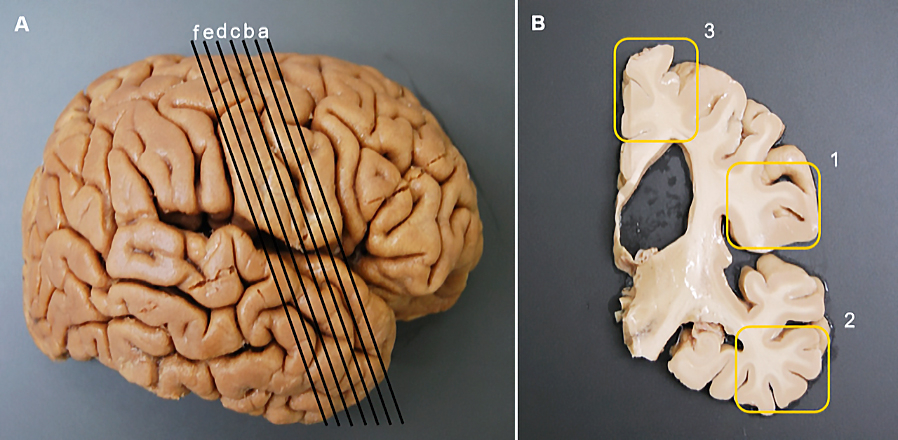
Fig. 1.
Brain sample preparation. Samples were obtained from 60 hemispheres. Each sample was cut in the coronal plane at 5-mm intervals anterior and posterior to the central sulcus (A). For the histological study, three sections (yellow box frames), one each from the 1) ventral operculum, 2) temporal lobe, and 3) dorsal operculum, were collected from each original slice (B).
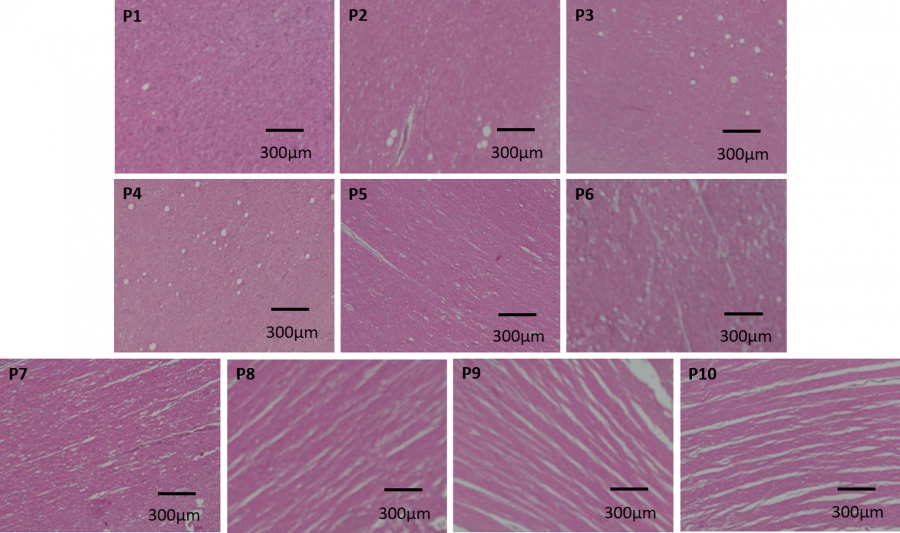
Fig. 2.
Hematoxylin-eosin (H&E)-stained brain samples that underwent different freezing protocols. Formalin-fixed (P1), 1-week continuous freeze (P2), 2-week continuous freeze (P3), 3-week continuous freeze (P4), 4-week continuous freeze (P5), 5-week continuous freeze (P6), two cycles of 1-week continuous freeze separated by a 6-hour thaw period (P7), three cycles of 1-week continuous freeze separated by 6-hour thaw periods (P8), four cycles of 1-week continuous freeze separated by 6-hour thaw periods (P9), and five cycles of 1-week continuous freeze separated by 6-hour thaw periods (P10). The degree of separation created by the formation of ice crystals between the axons is evident in the freeze-thaw protocols, particularly in the P8, P9, and P10 samples, while the freeze-only protocols (P2-P6) lack this separation.
Data processing
The images of the H&E-stained specimens were segmented and analyzed using the MATLABⓇImage Processing Toolbox TMapplication (MathWorks, Natick, MA, USA). Using the Image Segmenter application, white matter regions were selected and extracted from the scanned images (Fig.3B). The RGB colors of the digital images were converted into grayscale signals. Image contents were then categorized as either white matter fibers or gaps, where gaps were depicted in white (Fig.3C).
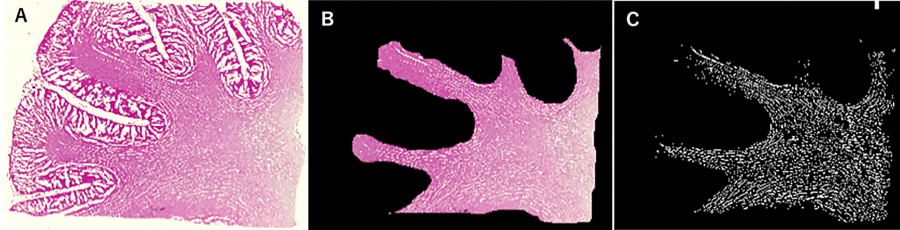
Fig. 3.
Slice image processing. Scanned images (A) were processed using the Image Segmenter software. White matter regions (B) and gap areas (C;white areas) were identified.
Assessment of specimens
Eccentricity is an anisotropy index. The mean eccentricity (e) of the gaps was measured to assess the direction of separation. The eccentricity of an ellipse is always between zero and one. An eccentricity of zero indicates an isotropic state (round shape) and as eccentricity approaches a value of one, anisotropy becomes significant (Fig.4). When an ice crystal separates the fibers along their axis, the eccentricity of the gap increases.
The ratio of total gap areas to white matter areas was also assessed to measure and evaluate the degree of fiber separation. The ratio was calculated by dividing the gap area (white area in Fig.3C) by the designated white matter area (Fig.3B).
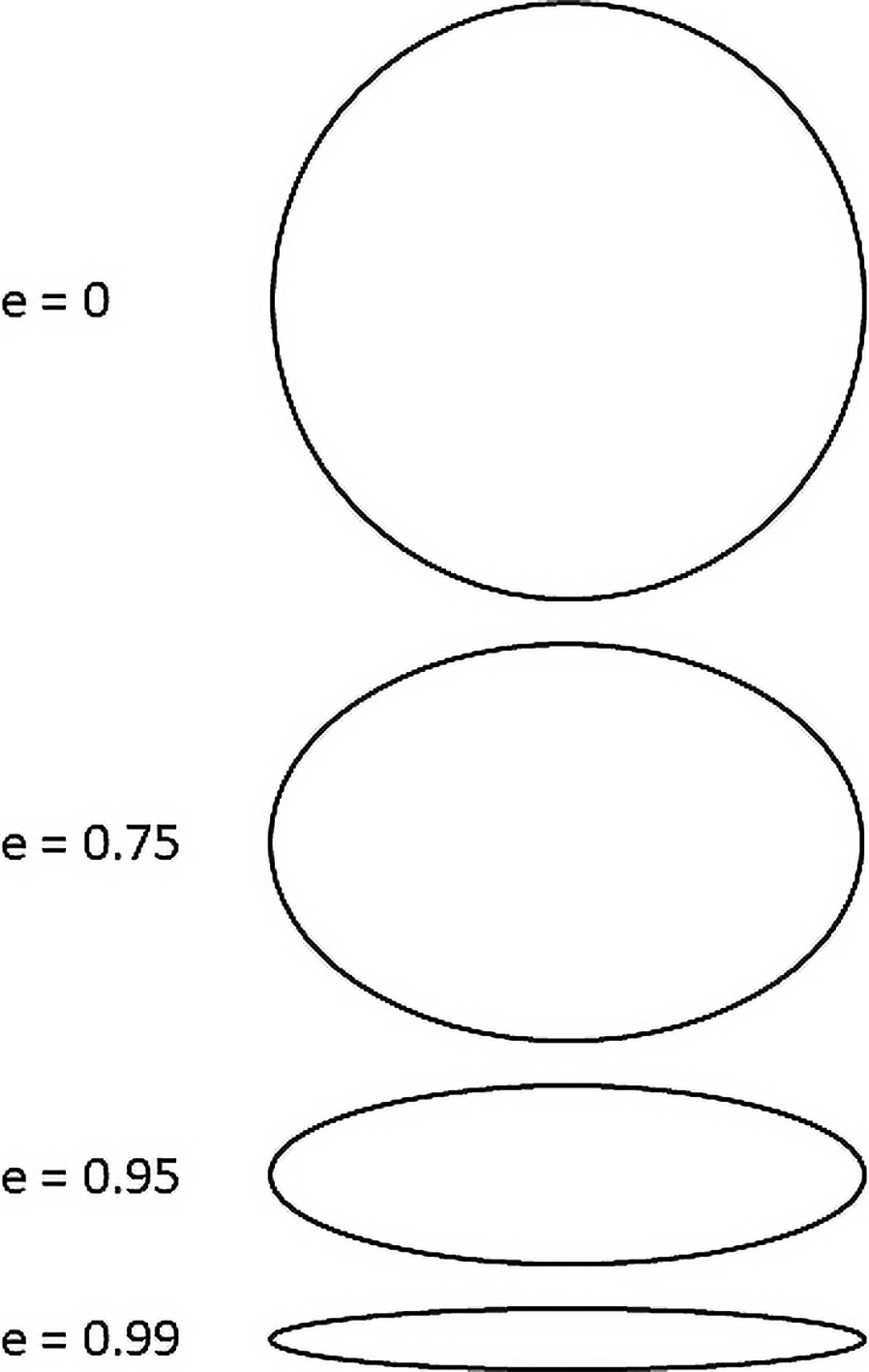
Fig. 4.
Eccentricity measurement. An eccentricity of zero is depicted as a spherical shape, which represents an isotropic state. As eccentricity increases, anisotropy also increases.
Statistical analyses
A standard one-way analysis of variance (ANOVA) was used to detect significant differences between the gap area ratios and eccentricity values between protocol groups. If the assumption of the equality of variance was violated, a Welch ANOVA was used. Specific significant results were then identified through a post hoc analysis of pairwise comparisons between all groups. If a Welch ANOVA was used, then the Games-Howell post hoc test was employed. First, differences in the frozen-only samples (P2-P6), including the formalin-fixed samples (P1), were analyzed, followed by the freeze-thaw samples (P7-P10), including the 1-week continuous freeze (P2) samples.
Data were checked for outliers, and normality was assessed using the Shapiro-Wilk test. Descriptive data are expressed as mean ± standard deviation, while results of the ANOVA and post hoc tests are expressed as mean ± standard error. Significance in all tests was set at p < 0.05. The JASP software, version 0.15, was used for all analyses.