The canonical function of HMGA2
The high mobility group (HMG) proteins are non-histone chromatin-associated nuclear proteins that regulate gene expression and chromatin structure34,35). Among three HMG superfamily members (HMGA, HMGB, and HMGN), the HMGA family consists of two members, HMGA1 and HMGA2. The HMGA family mainly functions to bind AT-rich regions in the minor groove of DNA36), change chromatin structures, and help DNA binding of TFs in cooperation with protein-protein interactions induced by the acid domain of HMGA237-40). HMGA2 is widely expressed in normal tissues during development, with expression levels decreasing in late development to adulthood41,42). Except for the development period, the role of HMGA2 in normal tissues is limited to the maintenance of stem cells and mesenchymal cells43-47). However, HMGA2 is re-expressed in various cancers and is associated with the progression of the disease48,49).
HMGA2 in myeloid malignancies
Numerous mechanisms of overexpression, roles in tumor progression, and target genes of HMGA2 have been reported in various cancers, and appear to be context-dependent48,49). In this review, we focus on the overexpression of HMGA2 in myeloid malignancies. A schematic cause-and-effect diagram of HMGA2 overexpression in myeloid malignancies is presented in Figure 1.
Overexpression of HMGA2 transcripts is reported in the whole blood and hematopoietic stem cell fractions of patients with MPNs, and the frequency of HMGA2 overexpression in patients with primary myelofibrosis (PMF), which is the most severe subtype of MPN, reaches nearly 100%50-55). In addition, overexpression of HMGA2 has been reported in some patients with myeloid malignancies such as chronic myeloid leukemia (CML)56-58), MDS59), AML56,60), as well as paroxysmal nocturnal hemoglobinuria (PNH)61,62), which is a benign but acquired clonal hematologic disease.
HMGA2 has a long 3’UTR, and it is targeted by various microRNAs (miRNAs)49,63). Disruption of miRNAs is implicated in the development and progression of hematopoietic malignancies as well as other cancers64,65). Among these miRNAs, family members of miR-Let7 are the most powerful degraders of HMGA2, as there are eight predicted miR-Let7 target sequences on the 3’UTR of HMGA266,67). Reduced expression of miR-Let7 and other microRNAs targeting HMGA2, or deletion of the 3’UTR of HMGA2, which includes target sequences of microRNAs, are implicated in overexpression of HMGA2 in hematological diseases53,54,62,68-75).
Also, LIN28B, the negative regulator of miR-Let7, is frequently overexpressed in progressive cancers76), and activation of the LIN28B-Let7-HMGA2 axis contributes to the progression of various cancers77). The LIN28B-Let7-HMGA2 axis is also an essential regulator in the development of hematopoiesis but is inactivated in adulthood78,79). Reactivation of this pathway in HSPC could be the cause of malignant transformation, but this requires further evidence about any relationships between LIN28B and myeloid malignancies.
Moreover, abnormal splicing contributes to overexpression of HMGA2 in patients without genetic amplification or translocation in HMGA2 coding lesion53,59,61), indicating that mutations or dysfunction of splicing factors may be associated with dysregulation of HMGA2 expression.
In addition to the mechanisms mentioned above, mutations in epigenetic modulators are associated with overexpression of HMGA2 in patients with MPNs55). MPNs are derived from HSPCs with constitutive activation of JAK-STAT signaling, which is provoked by driver mutations such as JAK2-V617F80,81), MPL-W515L/K82), and CALR-exon9-indels83,84). EZH2 mutation is one of the most frequent co-occurring mutations in JAK2-mutated MPNs and is associated with poor prognosis85,86). EZH2 is the catalytic component of the polycomb repressive complex 2 (PRC2), and loss of EZH2 is associated with overexpression of HMGA287,88).
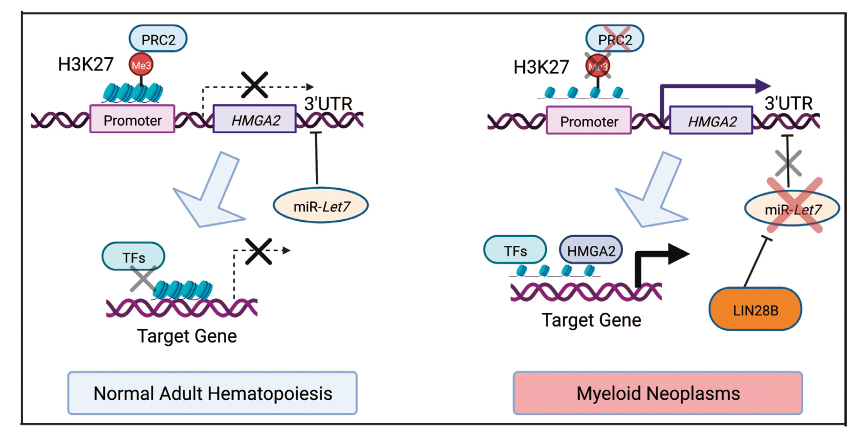
Fig. 1. The implication of HMGA2 on myeloid malignancies.
Schematic cause-and-effect diagram of HMGA2 overexpression in myeloid malignancies. In normal hematopoietic cells, expression of HMGA2 is repressed mainly by PRC2 via H3K27 tri-methylation of its promoter and/or miR-Let7-mediated silencing. Those suppressors are altered in myeloid malignancies leading to overexpression of HMGA2 followed by upregulation of its target genes. (Me3: tri-methylation, PRC2: polycomb repressive complex 2, TFs: transcription factors, UTR: untranslated lesion)
Murine models with HMGA2 overexpression
To clarify how HMGA2 contributes to disease progression, we and other groups generated several murine models that express external Hmga2 transgenes89,90) or re-express endogenous HMGA2 by deletion of Ezh287,88,91). A model of transgenic mice with a truncated murine Hmga2 (Hmga2-Tg, also described as ΔHmga2) shows about 3- to 5-fold increased expression of HMGA2 compared to wild-type (WT) controls in hematopoietic tissues. This model shows moderate elevation of white blood cell (WBC), red blood cell (RBC), and platelet (PLT) counts, increased number and repopulating capacity of HSPCs as well as increased megakaryopoiesis; however, it does not develop lethal myeloid disease89). Hmga2 conditional knock-in mice reported from elsewhere were phenotypically similar to those with Hmga2-Tg90). Of note, another model of HMGA2-Tg mice that overexpresses human HMGA2 has been reported to develop very aggressive acute lymphoid leukemia92). This might reflect the “context-dependent” oncogenic activity of HMGA2. Differences in species, promotors, and expression levels may lead to differences in which hematopoietic lineage would be the most affected.
Endogenous overexpression of HMGA2 by deletion of the PRC2 component Ezh2 has been reported in MPN models, which harbor the Jak2-V617F mutation. These mice developed lethal myelofibrosis, and RNAseq/ChIPseq of HSPC showed elevated expression of Hmga2 provoked by decreased H3K27 trimethylation in a promotor lesion87,88). To confirm that HMGA2 is responsible for disease progression in JAK2-V617F hematopoiesis, we crossed JAK2V617F-Tg mice93) with Hmga2-Tg89) (JAK2VF/Hmga2). JAK2VF/Hmga2 mice reproduced the phenotypes of JAK2VF/Ezh2-/- mice and died with severe leukocytosis. Proliferated leukocytes were mature, and did not represent AML. Myelofibrosis was observed, but mice with JAK2VF alone also presented with severe myelofibrosis, so we could not determine whether overexpression of HMGA2 promotes fibrosis. HSPC of JAK2VF/Hmga2 mice showed hematopoietic repopulating capacity after 3 iterations of competitive serial bone marrow transplantation, while JAK2VF alone was outcompeted by a WT competitor. Also, abnormal blood cell counts in JAK2VF/Ezh2-/- mice were partially recovered by a heterogenous knockout of Hmga2. We identified several oncogenes, Lmo1, Gcat, and Prss16 as the upregulated genes in HSPC of both JAK2VF/Hmga2 and JAK2VF/Ezh2-/-. However, upregulation of myelofibrosis-related pathways such as TGFβ signaling was observed only in JAK2VF/Ezh2-/-. Therefore, we concluded that HMGA2 contributes to the expansion of a JAK2-V617F mutated clone, and fibrosis is provoked by other targets of EZH255). However, this last point is still controversial as other groups showed that overexpression of HMGA2 upregulates TGFβ signaling, which plays a critical role in bone marrow fibrosis94,95), via overexpression of Tgfbr296). They employed another Jak2-V617F mouse, and overexpressed Hmga2 by lentivirus. Collectively, although its role in the progression of bone marrow fibrosis is controversial, we can conclude that HMGA2 enhances the fitness of JAK2-mutated clones, leading to disease progression (Figure 1).
Recently, HMGA1 – the sister protein of HMGA2 – has been reported to contribute to the progression of MPN. The investigators’ murine model revealed that heterogeneous loss of Hmga1 markedly improved myelofibrosis of JAK2-V617F-Tg mice97). They reported that targets of HMGA1 are proliferation pathways and GATA2, which is distinct from targets of HMGA2. This may suggest close collaboration between these sister genes in the pathogenesis of MPN and warrants further study.
Functional analysis of the CALR mutant, which is the second most frequent driver mutation in MPNs, has been insufficient, especially regarding its collaboration with other mutations. Recently, we generated Calr-del10 mice that lack 10 base pairs in exon 9 of Calr, mimicking type2-like CALR mutation in MPN patients; these mice presented mild phenotypes of MPN98). Our preliminary data have shown that addition of Hmga2-Tg to Calr-del10 evokes progression of MPN phenotypes but not myelofibrosis or leukemic transformation, while deletion of Ezh2 can provoke myelofibrosis or leukemic transformation after a long latency. From these observations, we speculate that HMGA2 just contributes to clonal expansion of MPN, and other targets of EZH2 contribute to myelofibrosis and clonal expansion, although this warrants further study.
Implications of HMGA2 in AML
Although HMGA2 is overexpressed in some of the patients with myeloid malignancies other than MPN56-60), the role of HMGA2 in these diseases remains uncertain. Our model showed that HMGA2 expands JAK2-mutated clones and provokes lethal MPN, however, JAK2VF/Hmga2 mice never develop overt AML55). A recent report showed that forced expression of Hmga2 in Tet2-deficient HSPC activates IGF2BP2 and its targets, and transplantation recipients of these cells develop lethal MDS but not overt AML99). These findings suggest that overexpression of HMGA2 is insufficient for a complete transformation to AML. HMAG2 may be associated with a leukemia stem cell (LSC) signature rather than a leukemic transformation. AML cells with high HMGA2 expression have been reported to present more immature surface markers and higher LSC scores compared to HMGA2-low AML cells100). Immature phenotype should be associated with worse prognosis, therefore inhibition of HMGA2 may improve the treatment of AML.
HMGA2 inhibitors
So far, no clinical-grade HMGA2 inhibitor is available. Netropsin is a pan-AT hook-binding drug that has been used experimentally101), but cannot be administered to humans. Ciclopirox and tetrac are reported as direct inhibitors of HMGA2102,103). Small molecule inhibitors of LIN28 have also been tested, and are expected to induce an abundance of Mir-Let7, leading to degradation of HMGA2104). Further study is needed to develop selective HMGA2 inhibitors for human therapy.
Summary
In summary, HMGA2 is inadequately re-expressed by genetic amplification, loss of regulatory lesion of 3’UTR, alteration of the LIN28B-Let7-HMGA2 axis, loss-of-function mutation in EZH2, or disruption of splicing (Figure 1). Overexpression of HMGA2 contributes to the expansion of disease clones harboring driver mutations of myeloid malignancies by activating genes involved in stem cell signatures.