Tau PET ligands
First-generation:
The radioligand 2-(1-{6-[(2-[Fluorine-18]fluoroethyl)(methyl)amino]-2-naphthyl}-ethylidene)malononitrile (18F-FDDNP) is the first PET tracer to visualize AD pathology in living humans14). 18F-FDDNP binds to both NFTs and amyloid plaques in the brain. In the first longitudinal PET study comparing tracer binding of 11C-PiB and 18F-FDDNP in AD patients, MCI patients, and healthy controls, 18F-FDDNP successfully discriminated AD from healthy controls with a 9-fold lower specific binding signal, possibly due to both amyloid and tau, compared to 11C-PiB. Interestingly, the development of 18F-FDDNP has opened the door to PET imaging of not only amyloid but also tau in other tau diseases. This is because postmortem histopathological studies have consistently demonstrated that NFT is a better indicator of disease severity and progression than Aβ.
One of the earliest agents developed for tau imaging was 11C-labeled PBB3, a pyrinated phenyl- and pyridinyl-butadienyl-benzothiazole with a 50-fold higher affinity for tau than Aβ deposits15). 11C-PBB3 is a PET tracer used clinically for in vivo detection of tau inclusions in AD as well as non-AD tauopathies in the human brain. However, its high white matter uptake, low target-to-white matter ratio in autoradiography of AD tissue, rapid in vivo metabolism, photoisomerization upon fluorescent light exposure, and brain-penetrating metabolites that make ligand uptake difficult to quantify have hindered its utility16). It also exhibits high retention in the dural venous sinus. Finally, the short half-life of 11C- labeling limits the availability of PET ligands compared to 18F labeling.
Tohoku University has developed a series of quinolone derivatives, including 18F-THK511717) and 18F-THK535118). Of the two, 18F-THK5351 has good imaging properties and is characterized by high uptake into gray matter, low uptake into white matter, and low lipophilicity. It shows high affinity for the tau protein isoform with four repeats in the microtubule-binding domain (4R-tau). However, high off-target binding of 18F-THK5351 was observed, especially in the thalamus, where blocking studies with the monoamine oxidase (MAO) B inhibitor selegiline showed a 40% drastic decrease in 18F-THK5351 uptake19). Cortical uptake was also significantly reduced due to this off-target binding, indicating that 18F-THK5351 cannot accurately quantify human tau levels in vivo.
The radioligand 7-(6-[18F]fluoropyridine-3-yl)-5H-pyrido[4,3-b]indol, also known as 18F-flortaucipir, 18F-AV1451, or 18F-T807, is the most extensively studied first-generation tau PET tracer20). In 2020, it was approved by the U.S. Food and Drug Administration as TauvidTM for PET imaging of adult patients with cognitive impairments undergoing evaluation for Alzheimer’s disease (AD) based on tau pathology21,22). The radioligand has high affinity for 3R/4R tau isoforms in AD patients. Autoradiography studies using human brain tissue samples from multiple neurodegenerative disorders have confirmed that 18F-flortaucipir binds specifically to paired helical filaments, tau-containing NFTs, and dystrophic neurites in AD brains20,23). However, there is little or no binding of 18F-flortaucipir to tau aggregates composed of straight filaments, as well as to alpha-synuclein or TDP-43 deposits. Off-target binding is observed in the choroid plexus24,25). This finding is of particular interest, as the choroid plexus is a critical area in the study of AD tauopathy, given its proximity to the hippocampus and its potential involvement in a critical stage of AD tauopathy progression. The choroid plexus is a dense collection of capillaries in an ependymal stroma surrounded by a layer of epithelium. Several substances found in the choroid plexus could potentially bind to 18F-flortaucipir, including melanin, which is supported by the fact that Black/African American individuals show higher tracer accumulation in the choroid plexus than White American individuals26). Other phenomena found in the choroid plexus that may accumulate 18F-flortaucipir include calcification/mineralization24), Biondi rings27), and iron deposits28). On the other hand, tangle-like structures of the epithelial cells in the choroid plexus that can be labeled with 18F-flortaucipir may also immunoreact to tau-specific antibodies29). This off-target binding should be interpreted with caution, as it primarily affects the measurement of 18F-flortaucipir accumulation in the hippocampus.
MAOs have been identified as a potential source of off-target binding in 18F-flortaucipir PET imaging. Vermeiren et al.30) reported that 3H-AV1451 binds with high affinity to either MAO-A or MAO-B, depending on the region of the brain being examined. Specifically, the binding of 3H-AV1451 in the temporal cortex is sensitive to clorgyline but not selegiline, indicating that most binding is to MAO-A. Conversely, in the thalamus and subthalamic nucleus, 3H-AV1451 binds equally to selegiline and clorgyline and to MAO-B, consistent with the known relative distribution and abundance of these two enzymes in the human brain. Although 3H-AV1451 has about 10-fold lower affinity for MAO-B than for MAO-A, the levels of MAO-B in the human brain are 2- to 10-fold higher than those of MAO-A, offsetting the lower affinity for MAO-B. However, in a study by Hansen et al.31), there were no significant differences in 18F-flortaucipir uptake between Parkinson’s disease patients who received MAO-B inhibitors and those who did not. This suggests that the use of MAO-B inhibitors at pharmaceutical levels does not significantly affect 18F-flortaucipir binding, and that MAO-B is not a significant binding target for 18F-flortaucipir in clinical studies.
Second-generation:
The second-generation tau PET ligands appear to have overcome several limitations associated with MAO off-target binding, which were observed in first-generation ligands32). For instance, 18F-RO948 exhibits low lipophilicity and a relatively low plasma-free fraction. Autoradiographic studies show that it binds with high affinity to tau aggregated in AD brain sections, while exhibiting lower reactivity in non-AD tauopathies. This suggests that 18F-RO948 primarily recognizes a mixture of 3R and 4R tau isoforms33). Unlike 18F-flortaucipir, 18F-RO-948 has excellent kinetic properties and appears to be free of off-target retention in the basal ganglia, thalamus, and choroid plexus34). Additionally, it lacks affinity for MAO-A and -B. However, Kuwabara et al.35) reported that off-target 18F-RO948 retention was observed in the substantia nigra and the cerebellar vermis in two young control individuals, which could be attributed to its binding to neuromelanin deposits.
Similarly, 18F-GTP1 exhibits high affinity and selectivity for tau pathology with no measurable binding to Aβ plaques or MAO-B in AD tissues or binding to other tested proteins at an affinity predicted to impede image data interpretation. In humans, it exhibits favorable dosimetry and brain kinetics, and no evidence of defluorination. Moreover, 18F-GTP1-specific binding is observed in cortical regions of the brain predicted to contain tau pathology in AD, and exhibits low test-retest variability36,37).
18F-PI2620 is structurally similar to 18F-flortaucipir, suggesting similar binding preferences to 3R/4R tau. Specific binding to pathologically misfolded tau was demonstrated by autoradiography on AD brain sections, whereas no specific tracer binding was detected on brain slices from non-demented donors. In addition to its high-affinity binding to tau aggregates, the compound showed excellent selectivity with no off-target binding to Aβ or MAO-A/B38). Elevated tau PET signal using 18F-PI2620 was observed in the medial temporal lobe and posterior cortical association areas in typical Aβ+ amnestic MCI and AD dementia39).
The [18F]-JNJ-64326067-AAA (18F-JNJ067) has high binding to tau and low binding to Aβ. AD participants showed elevated tracer relative to controls in the entorhinal cortex40). However, off-target signal in the putamen, pallidum, thalamus, midbrain, superior cerebellar gray, and white matter was observed. Lack of binding in HCs, MCIs, and PSPs suggests that 18F-JNJ067 may not bind to low levels of AD-related tau or 4R tau.
18F-PM-PBB3, APN-1607, also known as 18F-florzolotau, is a propylated analogue of 11C-PBB3. It was proposed to circumvent the off-target binding of 11C-PBB3, metabolic instability, and the brief half-life of 11C- compared with 18F. Unlike 11C-PBB3, there was no sign of off-target binding in the basal ganglia and thalamus, while there was no sign of binding to MAO. PM-PBB3 had more distinct binding in the choroid plexus41,42). PM-PBB3 can recognize tau pathologies in both AD and non-AD brains, including progressive supranuclear palsy41), corticobasal degeneration43), and Pick’s disease44). Autoradiography studies of frontal AD brain homogenates showed that 18F-PM-PBB3 has a high binding potential to tau without binding to MAO enzymes and little to Aβ in vitro41). However, other in vitro studies45) demonstrated significant relationships of APN-1607 with amyloid plaque pathology. Co-incubation of ALS patient frontal cortex and motor cortex tissues with 3H-APN-1607 and 10μM amyloid-specific agent NAV-4694 was carried out to define the contribution of Aβ to the radioligand signal and revealed an average inhibition of 25.0±20.4% gray matter. Additionally, off-target binding to α-synuclein fibrils by the parent molecule, 11C-PBB3, and derivatives has been described46,47). These studies suggest that APN-1607, the parent compound 11C-PBB3, and derivatives may display binding to a number of pathological proteins.
The radioligand, 6-[18F]fluoro-3-(1H-pyrrolo[2,3-c] yridine-1-yl) isoquinolin-5-amine (18F-MK6240)48) exhibited low binding to MAO-B in molecular docking studies49). Nonhuman primate blocking studies also showed no apparent off-target binding50). Several studies have established that the binding patterns of this ligand are associated with NFT deposition in AD51-53). Conversely, 18F-MK-6240 does not bind to the entorhinal cortex of the non-AD brain50). The tracer displays favorable kinetics with rapid brain delivery and washout without labeling of the white matter48,50). The cerebellar gray matter has low binding across individuals, demonstrating its potential use as a reference region. A reversible two-tissue compartment model well described the time-activity curves across individuals and brain regions. Initial 18F-MK6240 PET studies revealed distribution volume values above 4 in the AD cortex, indicating high affinity to NFTs in vivo, accompanied by a low non-displaceable signal52). Off-target binding regions included the retina, ethmoid sinus, clivus, meninges, and substantia nigra, but not the basal ganglia or choroid plexus54). These off-target bindings are related to melanin and neuromelanin-containing cells55). The standardized uptake value ratio (SUVR) of 18F-MK6240 was two to four in NFT-rich regions of AD patients 60-90 minutes after tracer injection, indicating high uptake, while the SUVR was around one throughout healthy control brains53). Across all subjects, the tracer exhibited adequate test-retest variabilities for various endpoints in NFT-rich brain areas56). The binding pattern of 18F-MK6240 in target areas of amyloid-positive patients without dementia and AD patients correlated with Braak staging 57,58) of NFT accumulation52,54). A direct comparative study59) with 18F-flortaucipir demonstrated complete concordance of visual ratings between the two radiotracers for both the medial temporal lobe and the neocortex, while the dynamic range of SUVR in target regions was approximately two-fold higher for 18F-MK6240 than for 18F-flortaucipir. This greater dynamic range for 18F-MK6240, with much less off-target binding than for 18F-flortaucipir, may be an advantage in detecting early tau pathology or in performing longitudinal studies to detect small interval changes.
Visual interpretation of tau PET
For visual interpretation of tau PET, second-generation tracers with less off-target binding are preferable compared to amyloid PET. This is because amyloid PET may have difficulty detecting mild accumulation in the targeted cortex due to nonspecific diffuse white matter accumulation. Visual interpretation of tau PET can be improved by using neuropathological NFT staging instead of a simple positive or negative classification. According to Braak staging, tau propagation can be classified into six stages based on the location of the tangle-bearing neurons and the severity of changes57,58). The first two stages, characterized by preferential involvement of the transentorhinal region and mild hippocampal involvement, are known as the “transentorhinal stages.” The next two stages, marked by significant involvement of both the entorhinal and transentorhinal regions, mild to moderate hippocampal involvement, and low neocortical involvement, are called the “limbic stages.” The final two stages, characterized by devastating neocortical involvement, are known as the “neocortical stages.” Pascoal et al.54) suggested using 18F-MK6240 Braak stages based on this neuropathological NFT staging. This staging method can help stratify individuals with abnormal tau deposition in clinical and research settings. An 18F-MK6240 Braak stage of 0 indicates a low risk of amyloid-β pathology, neurodegeneration, and forthcoming cognitive impairment. An 18F-MK6240 Braak stage of IV or above indicates a high risk of underlying neurodegeneration, and an 18F-MK6240 Braak stage of V-VI is associated with impending onset of dementia.
On the other hand, Shuping et al.60) proposed four visual read classifications for 18F-MK6240: “no uptake,” “MTL only,” “MTL AND,” and “outside MTL.” “No uptake” is defined as a lack of elevated 18F-MK-6240 signal in medial temporal or neocortical regions, or signal in any “off-target” brain region such as the striatum, cerebellum, or midbrain that does not exceed the signal in the retina. “MTL only,” corresponding to 18F-MK6240 Braak stages I-II, is defined as elevated intensity in any MTL structure, including the transentorhinal, entorhinal, subiculum, hippocampus, parahippocampus, and amygdala, in either hemisphere, without any neocortical uptake. “MTL only” identifies this uptake while distinguishing it from neocortical spread associated with amyloid-dependent disease progression61,62). This MTL only accumulation is also observed in primary age-related tauopathy63,64). The sagittal slice of PET in AD shows a “hook-like” uptake of strong accumulation in the amygdala and entorhinal area to the parahippocampus and weak accumulation in the hippocampus65) (Fig. 1a). “MTL AND,” corresponding to 18F-MK6240 Braak stages III and above, indicates elevated signal in MTL and at least one additional neocortical region, in either/both hemispheres. Uptake in the lateral temporal neocortex, including anterolateral tissue and fusiform, in frontal, parietal, occipital, and/or cingulate regions all contribute to “AND.” “MTL AND” captures neocortical NFT spread associated with AD progression and an accelerated rate of NFT accumulation, the slowing of which is the goal of several clinical trials. This spatial discrimination is relevant to monitoring disease progression and treatment response. “Outside MTL” is defined as uptake in neocortical regions or in subcortical regions other than MTL that exceed retinal intensity. Some scans show focal uptake only in areas with a clinical phenotype different from typical AD, such as the occipital and frontal areas, and cases that show a medial temporal preservation pattern with neocortical uptake typical of AD. Non-brain off-target signal, such as meninges, does not constitute positive uptake.
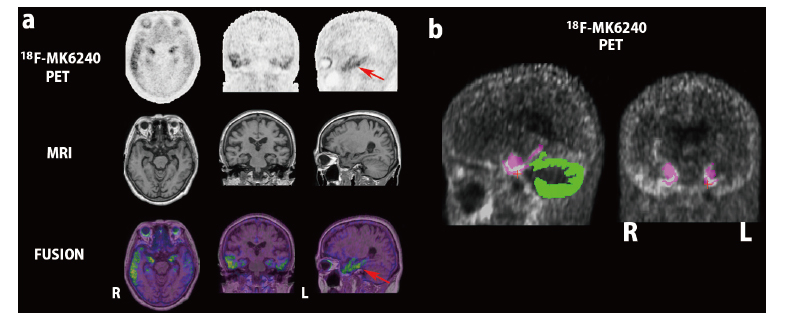
Fig. 1. Tau PET using 18F-MK6240 in a woman with AD in her early 70s
The present study was approved by the certified Clinical Research Review Board at the National Institutes for Quantum Science and Technology, and all subjects or their legal representatives gave written informed consent. This study was registered in the Japan Registry of Clinical Trials (jRCTs 031210318).
a. Visual interpretation with classification of “MTL AND” at Braak stage IV.
Tau accumulation in MTL showed “hook-like” pattern in sagittal sections of PET and FUSION images (arrows).
b. SUVR measures using FreeSurfer parcellation.
Right and left mesial temporal masks (pink) showed SUVRs of 2.21 and 1.69, respectively with a reference mask of entire cerebellar gray matter (green).
Quantitative analysis of tau PET
As an adjunct to visual interpretation, quantitative analysis of tau PET has been proposed. However, the significance of quantitative analysis of tau PET in the brain is still in the research stage, and its clinical significance remains to be determined. Establishing a quantitative measurement would contribute to a more objective and longitudinal evaluation, as well as determination of the therapeutic effects of AD-modifying drugs currently under development.
Villemagne et al.66) constructed three regional tau masks: mesial-temporal (Me), which comprises the entorhinal cortex, hippocampus, parahippocampus, and amygdala; temporoparietal (Te), which comprises the inferior and middle temporal, fusiform, supramarginal, and angular gyri, orbitofrontal cortex, gyrus rectus, posterior cingulate/precuneus, superior and inferior parietal, and lateral occipital; and the rest of the neocortex (R), which comprises the dorsolateral and ventrolateral prefrontal, superior temporal, and anterior cingulate. A global measure of neocortical tau was calculated from the average of the Te and R composite regions.
These tau masks are usually sampled from 3D T1-weighted MRI images that are coregistered to tau PET using MRI-based native space FreeSurfer parcellations67) (Fig. 1b). Studies have also reported using the inferior cerebellar gray matter as a reference region instead of the entire cerebellar gray matter to avoid contamination from the adjacent cerebellar tentorium and to more accurately estimate standardized uptake value ratio (SUVR)52,67). Furthermore, the Centiloid scale is expected to be used as a harmonized value for standardizing each analytical method or PET ligand used, as in amyloid PET68).