Of the 407 infants enrolled in the present study, we excluded 39 infants (nine were transferred to other hospitals, three had unknown gestational ages, one died on day 0 due to severe respiratory distress syndrome, two had trisomy 21, one had hemophilia A, and 33 had missing information); the remaining 358 infants were included in the analysis (Figure 1). We performed a complete case analysis. The patient characteristics are shown in Table 1. The infants were delivered at a median gestation period of 34.4 weeks (interquartile range [IQR], 33.3-35.3 weeks), and their average birth weight was 1,993 ± 397 g. Moreover, 21% of the infants (n = 75/358) were categorized as showing an SGA status, 23% (n = 84/358) had a twin birth, and 75% (n = 269/358) were born via cesarean delivery. The Hb at birth was 16.9 ± 2.2 g/dL, and the Ret at birth was 25.1 ± 6.5 × 104/μL. Among the included children, 44% (n = 158/358) were treated for AOP. The Hb at treatment initiation was 12.6 ± 1.5 g/dL, and the corresponding values at the initiation of EPO and iron supplementation were 13.0 ± 1.3 g/dL and 12.7 ± 1.5 g/dL, respectively.
Results of the univariable analysis and multivariable logistic regression model of the primary outcome and treatment of AOP are shown in Table 2. In the univariable analysis, gestational age (p <0.001), SGA status (p = 0.004), cesarean delivery (p <0.001), maternal Hb before birth (p = 0.005), high-intensity breastfeeding (p <0.001), number of large samplings (p <0.001), and number of small samplings (p <0.001) were significantly associated with the development of AOP. Laboratory data at birth, such as Hb (p = 0.03), MCV (p <0.001), MCHC (p = 0.03), and Ret (p = 0.007) were significantly associated with AOP treatment. Ht was excluded from the multivariable analysis because of its high multicollinearity (variance inflation factor > 10). In multivariable analysis, SGA infants required significantly higher administration of agents for AOP treatment (odds ratio [OR], 7.17; 95% confidence interval [CI], 2.15-23.9). Lower maternal Hb before birth (OR, 0.66; 95% CI, 0.49-0.87), infants with low Hb at birth (OR, 0.71; 95% CI, 0.57-0.89), and a higher number of large samplings (OR, 1.79; 95% CI, 1.40-2.29) also had significantly higher odds of receiving treatment for AOP. Cesarean delivery, treatment for jaundice, number of small samplings, MCV, MCHC, and Ret at birth were not significantly associated with the treatment of AOP. Results of the sensitivity analysis are shown in Table 3. Similar to the findings for the primary outcome, SGA, Hb at birth, and number of large samplings were significantly correlated with higher odds of minimum Hb <12 g/dL during hospitalization. On the other hand, maternal Hb before birth was not significantly correlated with higher odds of the outcome.
The results of multivariable logistic models for EPO administration and iron supplementation are shown in Table 4 and 5. Infants with an SGA status (OR, 3.67; 95% CI, 1.35-9.99) and a higher number of large samplings (OR, 1.42; 95% CI, 1.17-1.73) had significantly higher odds for administration of EPO. Infants with an SGA status (OR, 3.67; 95% CI, 1.51-16.1), low maternal Hb before birth (OR, 0.66; 95% CI 0.50-0.88), lower Hb at birth (OR, 0.75; 95% CI, 0.60-0.93), and a high number of large samplings (OR, 1.70; 95% CI, 1.34-2.16) showed significantly higher odds of iron supplementation. Thus, we selected gestational age, SGA status, maternal Hb before birth, Hb at birth, and number of large samplings as variables significantly associated with the primary outcome, and we used these five variables for the ROC analysis. The ROC analysis for the primary outcome is shown in Figure 2. The area under the curve was 0.953 for the development of AOP. The sensitivity and specificity at the cutoff point were 0.91 and 0.88, respectively. Results of the analysis of associations between development of AOP and duration of parenteral nutrition and daily weight gain are shown in Table 6. No significant association was observed among those factors.
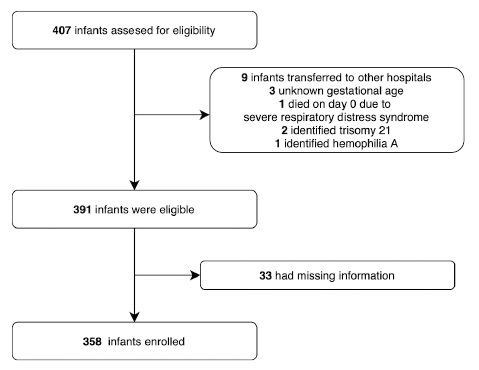
Fig. 1. Infant selection flow chart
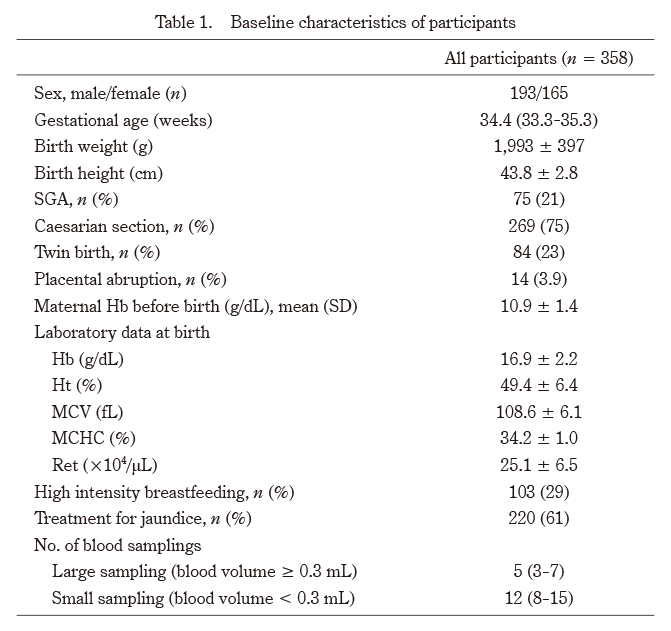
Table 1. Baseline characteristics of participants
Continuous data are expressed as median (interquartile range) for gestational age, large sampling, and small sampling or mean ± SD for other variables.
SGA, small for gestational age; Hb, hemoglobin; Ht, hematocrit; MCV, mean corpuscular volume; MCHC, mean corpuscular volume; Ret, absolute reticulocyte count
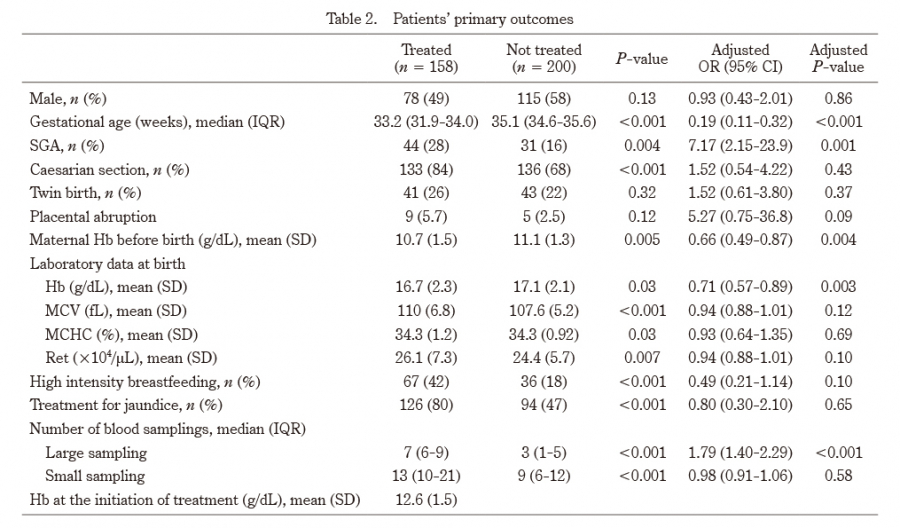
Table 2. Patients’ primary outcomes
SGA, small for gestational age; Hb, hemoglobin; MCHC, mean corpuscular hemoglobin concentration; MCV, mean corpuscular volume; Ret, absolute reticulocyte count
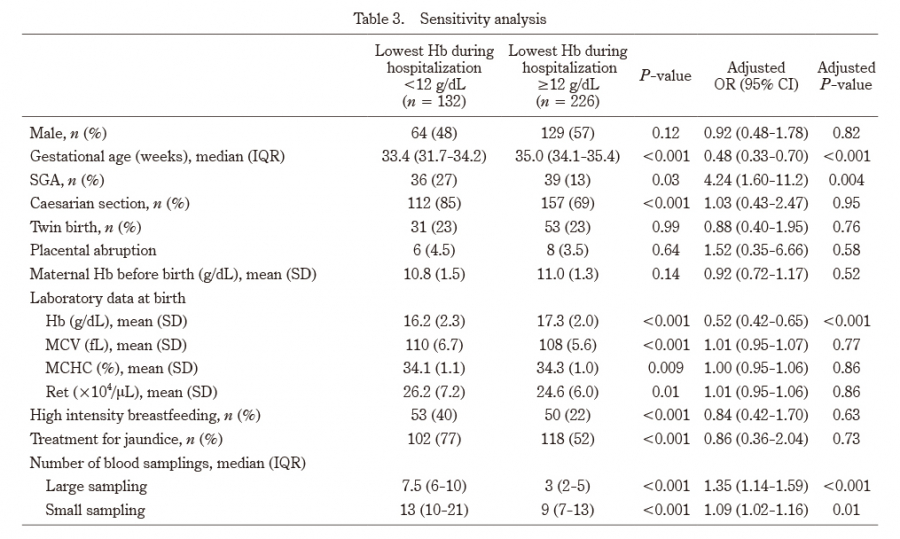
Table 3. Sensitivity analysis
SGA, small for gestational age; Hb, hemoglobin; MCHC, mean corpuscular hemoglobin concentration; MCV, mean corpuscular volume; Ret, absolute reticulocyte count
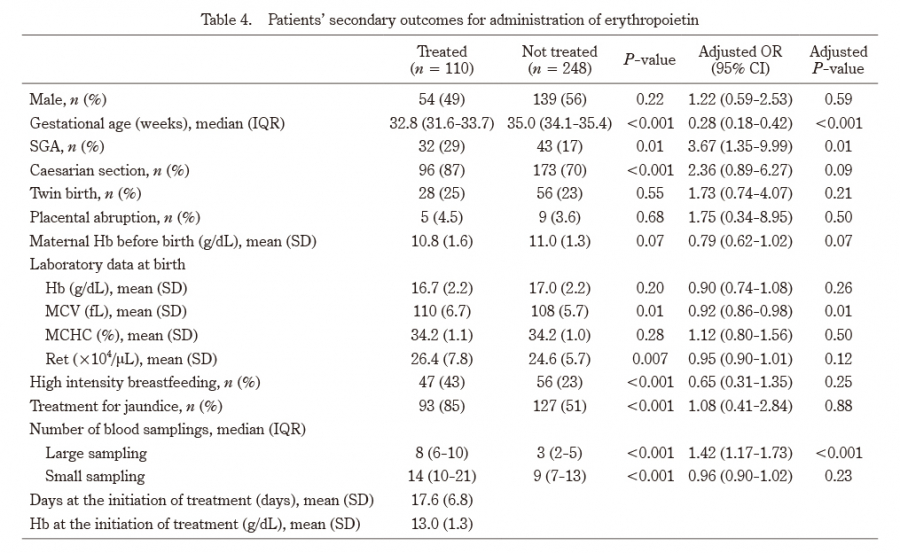
Table 4. Patients’ secondary outcomes for administration of erythropoietin
SGA, small for gestational age; Hb, hemoglobin; MCHC, mean corpuscular hemoglobin concentration; MCV, mean corpuscular volume; Ret, absolute reticulocyte count
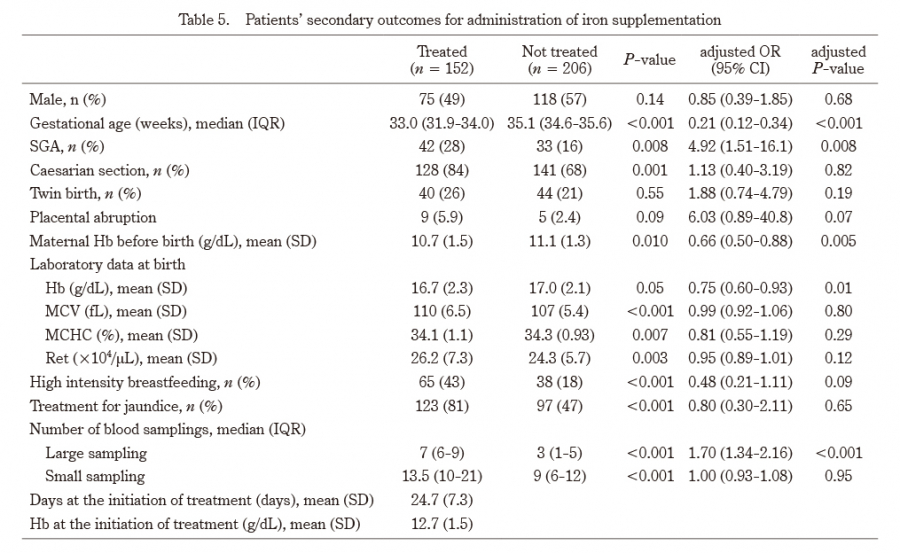
Table 5. Patients’ secondary outcomes for administration of iron supplementation
SGA, small for gestational age; Hb, hemoglobin; MCHC, mean corpuscular hemoglobin concentration; MCV, mean corpuscular volume; Ret, absolute reticulocyte count
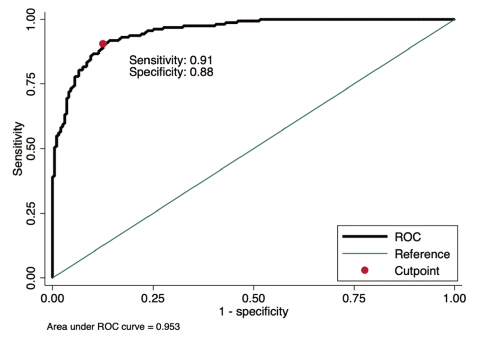
Fig. 2.
Receiver operating characteristic curve of the multivariable logistic regression model using statistically significant variables to detect the development of anemia of prematurity.
We selected gestational age, hemoglobin level at birth, small for gestational age or light for gestational age, last maternal hemoglobin level before birth, and number of large blood samplings as the variables. The cutoff point was investigated using Youden’s index test. The sensitivity and specificity at the cutoff point are shown.

Table 6. Association between treatment for anemia of prematurity and duration of parenteral nutrition or daily weight gain