Participants were 18 male first-division university baseball fielders (age 20.2±1.1years; height 1.75±0.05 m; mass 74.3±5.7 kg; baseball career 11.2±1.8 years). Of them, eight players bat right-handed and throw right-handed (44%), one bats left-handed and throws left-handed (6%), and nine bat left-handed and throw right-handed (50%). Participants in this study had no history of lumbar spine surgery or terminal-stage lumbar spondylolysis, and none of the athletes smoked. In addition, none of the participants had back pain at the time of motion analysis.
The study was approved by the Research Ethics Committee of the Faculty of Health and Sport Sciences, University of Tsukuba, Japan (approval number 27-118). After receiving a detailed explanation of the study, all participants signed informed consent forms.
Assessment of disc degeneration
Lumbar disc degeneration was evaluated by lumbar MR midsagittal imagery with a 0.25-T G-scan Brio (Esaote, Belgium). T2-weighted images (TR/TE = 2280/125, matrix = 256 × 252, thickness = 5.0 mm, FOV = 320 mm) were taken in the standing position (Fig. 1). Degeneration was assessed from L1/L2 to L5/S1 and was classified into 5 grades according to Pfirrmann’s classification16). As in Hangai et al.6) and Kaneoka et al.17), Grade III or higher was considered sufficient indication of degenerated discs (Fig. 1). In addition, we classified participants with disc degeneration at one or more disc levels as participants with disc degeneration. The MR images were interpreted by an orthopedic surgeon with abundant clinical experience.
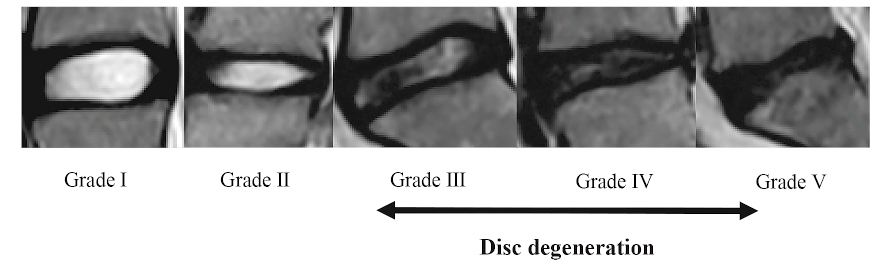
Fig. 1. Pfirrmann disc classification.
Degeneration was assessed from L1/L2 to L5/S1 and was classified into 5 grades according to Pfirrmann’s classification. As in Hangai et al. and Kaneoka et al., Grade III or higher was considered to indicate disc degeneration.
Motion analysis
Motion analysis was performed with the examiner and participants blinded to the MRI evaluation. Each participant wore a sleeveless shirt, tight shorts, socks, sneakers, and a cap during testing. Each participant had 47 markers attached to their body and 6 markers on the bat. The movement was tee batting with 3 swings each. The height of the tee was adjusted to the height of the participant’s belt, and the position was adjusted to the center of the base. Each participant was instructed to hit toward center field with his normal batting motion. For each participant, data from the swing with the fastest bat speed were used. The test-retest reliability (intraclass correlation coefficient [ICC]) between swing trials was very high (0.93). Three-dimensional (3D) coordinate data of the batting motion (body, 47 markers; bat, six markers) were captured using a 14-camera motion capture system (Vicon-MX, Vicon Motion Systems, Ltd., Oxford, UK) at 250 Hz (Fig. 2). Ground reaction forces of each leg were recorded on two force plates (9281A, 9287B; Kistler Instruments AG, Winterthur, Switzerland) at 1,000 Hz. The global coordinate system treated the subject’s left-right direction as the x-axis, the anterior-posterior direction as the y-axis, and the vertical direction as the z-axis.
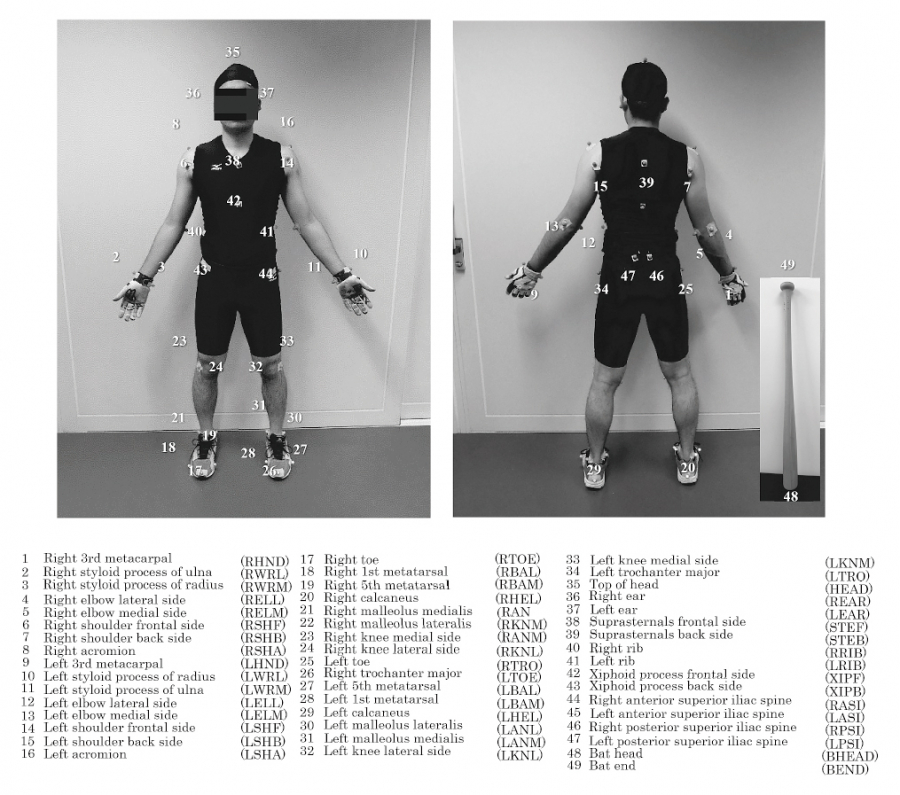
Fig. 2. Placement of the reflective markers on participants.
Data processing
We smoothed the 3D coordinate data of each subject with a second-order low-pass Butterworth digital filter to determine the optimal cut-off frequency (10.0-25.0 Hz) by the method described by Wells and Winter18).
The joint angle used in this study indicates the angle in the horizontal plane (x-y plane). The line between the right and left acromia projected into the horizontal plane was defined as the shoulder vector. The line between the right and left greater trochanters projected into the horizontal plane was defined as the hip vector. The line between the right and left anterior iliac spines projected into the horizontal plane was defined as the pelvis vector. Angles were compared between players with and without disc degeneration. The angle between the shoulder vector and the hip vector was defined as the torsion angle of the trunk (“twist”). The angular velocities of the shoulder line, hip line, pelvic line, and twist were calculated by time-differentiation of each angle. The swing speed of the bat head was calculated by time-differentiation from the obtained coordinate values.
We analyzed several features: shoulder, hip, pelvis, and twist; rotation angular velocity of the tee batting motion from foot release to follow-through; rotation angle and rotation angular velocity at the points of foot release, foot contact, take-back, maximum bat speed, and follow-through (Fig. 3); average angular velocities from foot release to foot contact, foot contact to take-back, take-back to maximum bat speed, and maximum bat speed to follow-through; the difference in time from foot contact to the maximum angular velocity of the shoulder line, hip line, and pelvic line; and the maximum angular velocities of the shoulder, hip, and pelvis.
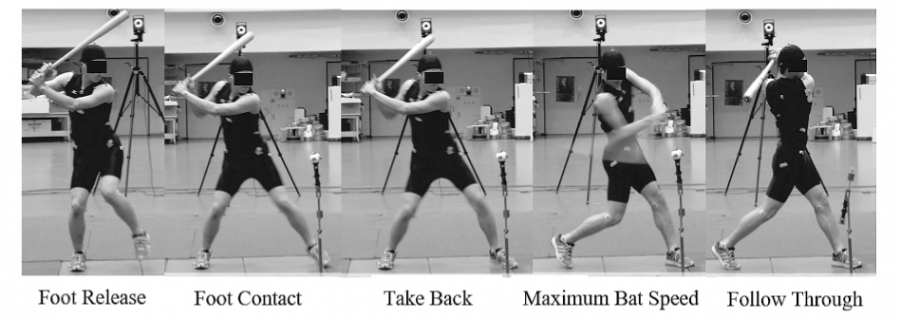
Fig. 3. Phases of baseball batting motion.
Foot release: immediately after the stepping leg is off the ground. Foot contact: when the stepping leg reaches the ground. Take-back: at the time of maximum twist angle at take-back.
Maximum bat speed: the highest speed at the top of the bat. Follow-through: time of maximum twist angle during follow-through.
Surface electromyogram analysis
A wireless electromyogram (EMG) sensor (Telemyo DTS, Noraxon Inc., USA) was used to record the surface electric potential from electrodes placed at the erector spinae (ES), multifidus (MF), latissimus dorsi (LD), gluteus medius (GMe), rectus abdominis (RA), external oblique (EO), and internal oblique (IO) muscles on both sides of the body. The skin was first exfoliated with a skin abrasive and alcohol-absorbent cotton to minimize artifacts.
Bipolar surface electrodes (Blue Sensor M-00-S/50, Ag/AgCl, Ambu A/S, Ballerup, Denmark) with a diameter of 34 mm were then placed on the muscle bellies and aligned 2 cm apart parallel to the muscle fibers according to the SENIAM recommendations for surface electromyography19). The derived myoelectric potential (MEP) was amplified by an EMG amplifier, The derived myoelectric potential (MEP) was amplified by an EMG amplifier, the signal was captured at a sampling rate of 1,000 Hz, pre-amplified at the source at a gain of 500 Hz, converted by 16-bit analog to digital converter. All records of myoelectrical activity were stored on a personal computer for later analysis. We measured the MEP at maximum voluntary contraction (MVC) of each muscle by adding maximum resistance using the manual muscle testing normal procedure of Daniels et al.20) the subject performed maximum isometric contraction for 3 s. The root-mean-square of stable 1s measurements was used as the action potential. The myoelectric waveforms were analyzed in MR3 Myomuscle software (Noraxon Inc.). The data were bandpass-filtered at 10 to 500 Hz to remove motion artifacts and then full-wave rectified in MR3 Myomuscle.
Data were analyzed from foot release to follow-through of the forefoot. The percentage (%) MVC was calculated as the value of the muscle action potential obtained during foot release, foot contact, take-back, maximum bat speed, and follow-through divided by MEP and used as the degree of muscle activity. An external analog output module was connected to the Vicon-MX to ensure synchronization with the surface EMG and optical signals.
Statistical analysis
Statistical analyses were performed in IBM SPSS Statistics Base 25 software. Unpaired t-tests were used to evaluate the differences between the two groups in terms of shoulder, hip, pelvis, and twist angles; angular velocities at points of foot release, foot contact, take-back, maximum bat speed, and follow-through; in average angular velocities from foot release to foot contact, foot contact to take-back, take-back to maximum bat speed, and maximum bat speed to follow-through; in time from foot contact to the maximum angular velocity of shoulder, hip, and pelvis; and the time difference in the maximum angular velocity between shoulder and hip and between shoulder and pelvis. Muscle activity did not show a normal distribution, so the Mann-Whitney U-test was used. Cohen’s d was calculated for the effect size of independent t-tests, with values of ≥ 0.20 and < 0.50, ≥ 0.50 and < 0.80, and ≥ 0.80 indicating a small, medium, and large effect, respectively21). Statistical significance was set at P < 0.05.